In diagnostic and cell sorting applications, microfluidics facilitate detection of pathogens or cells of interest. Sensors, usually optical detectors, are interfaced with a microfluidic cartridge for imaging or measuring a quantity of interest. With an optical detector, analytes can be characterized, quantified, classified (in case of cells and microparticles) and in some applications, sorted (such as in cell sorting).
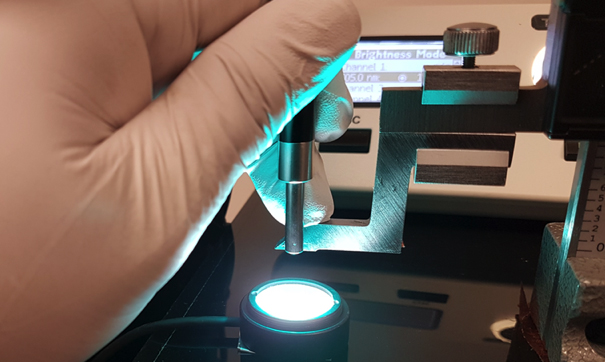
Major components of an optical detection module include a light source, lenses, mirrors, filters, cameras or light sensors, and a mechanism to focus or move the microfluidic interrogation window into position for alignment. Since the features of microfluidic cartridges are typically on the microscale (1um–1000um), microscope objectives are usually used.
In a traditional microfluidic optical system, most of the optical components reside in an instrument reader. Many off-the-shelf optics components are bulky and cannot be placed in a cartridge. The cartridge typically should be as simple as possible, however, in some designs lenses are built into a cartridge’s detection area (i.e., on-chip microfluidic optics systems).
Microfluidic Optical Detection Designs
A microfluidic optical detection system can be similar to a standard microscope. Such a design may be used to detect target cells or other objects of interest. The system shown in Figure 1 includes a light source, an optical filter, a lens, a focusing mechanism and an image capture device. The light source could be a lamp, a laser or an LED depending on the needed characteristics of the light (wavelength, power, etc.).
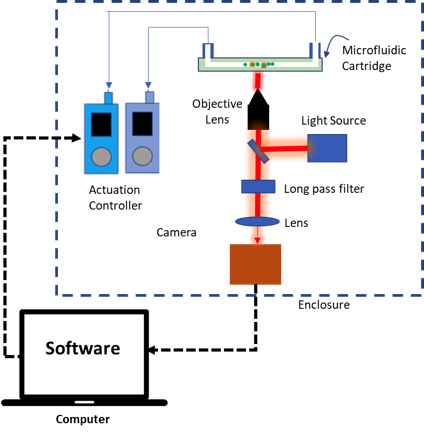
Lenses are used to magnify the sample and form an image on a camera sensor. An optical filter is used to isolate light in a particular wavelength range of interest. Lastly, an optical detector (i.e., a camera sensor) collects the light from the sample. Data is then captured from the detector and stored for further analysis. Similar microscope systems are very popular due to the availability of parts and the ease of coupling such systems to a microfluidic platform. In a microfluidic breadboard system, this type of set-up can be used for its simplicity and short time to build. However, such systems are difficult to miniaturize due to the optical path lengths involved.
To build an optical detection for microfluidic applications, the following guidelines are recommended.
- 1. The main goal of optical sensors is to detect target analytes in an assay. Hence, it is important to have a mature assay. Maturity means the assay has a proven protocol and has been tested using a commercially available instrument reader. Biochemical methods and appropriate fluorophores for labelling the target cell or microparticles should be known.
- A supplier of the appropriate fluorophores should be identified and excitation and emission wavelengths of these fluorophores should be noted.
- With the assay information known, an optical detection platform can now be designed and built. Create a high-level optical block diagram and identify the parts and their specifications.
- If required, use optical filters to isolate the wavelength range of interest to improve the identification and quantification of the target analytes. In applications that use fluorescent tags, one or more filters are typically used to block the light used to excite the samples, while passing the light emitted from the samples. Depending on the application and methods used, the instrument may have one or more filters for detecting multiple emissions.
- In an imaging system, carefully design and build a focusing mechanism. This is crucial in detecting the target labelled cells or microparticles. In a fluorescence assay to collect light in microfluidic detection channel, a focusing mechanism is necessary to increase the collected light. For portable point-of-care instruments, wherein the enclosure has constrained dimensions and footprint, the right placement and orientations must be taken into account in designing the focusing system. There is also a need to consider how the optical parts will be aligned in the system.
- For the image capture device, decide on the type and specifications of sensors to be employed (i.e. camera, PMT or Photodiode). Under low light conditions, a sensor with large pixels may be a good option due to the greater amount of light captured by each pixel (assuming a given irradiance such as optical power per unit area of light hitting the sensor). For small pixel size cameras, techniques such as pixel binning or averaging multiple images can be used to improve the quality of the collected data.
- Finally, test the newly built optical detection platform.
Most Common Microfluidics Imaging Techniques
Optical detection can be performed using a number of different detector types. The most common optical detectors used are PMTs, CCD sensors, CMOS sensors and photodiodes. Detectors integrated into microfluidic cartridges are usually photodiodes (silicon or organic-OPDs) or CMOS sensors (in some applications, CMOS sensors can even permit lens-free imaging).
In some cases, the optical and optoelectronic components are fully integrated within the microfluidic cartridge. Many device developers implement such designs due to their simplicity of use, sensitivity, integration into the manufacturing process, and ability to tune the optical properties on chips.
The applications for optical detection in microfluidics are widely recognized in analytical biochemistry (detection of proteins, cytometry, enzyme kinetics), point-of-care diagnostics, cell biology, and immunoassays, as well as in screening applications and fluid manipulation. The four most common optical properties measured are absorbance, fluorescence, chemiluminescence and surface plasmon resonance.
1. Absorbance
Absorbance measurements quantify the attenuation of incident radiation as light passes through a sample as a function of wavelength. Such measurements are a common and well-established technique for biological and chemical analysis in a microfluidic cartridge format. It is a simple, quick, and robust method of detecting the presence of the target analyte within a fluid sample, provided the analyte concentration is relatively low and the sample absorbs in an accessible wavelength range.
Incident light passing through a sample will attenuate by an amount that depends on the molecular absorbance spectrum of the sample, the spectrum of incident light, the concentration of analyte in the sample, the path length of light through the sample, and scattering of light from the sample. The setup to measure absorbance is relatively simple, but tends to have low sensitivity. Figure 2 illustrates a microfluidic layout showing a microfluidic cartridge in an absorbance measurement setup.
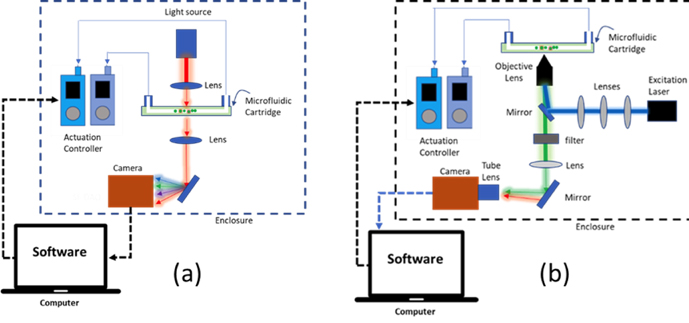
2. Fluorescence
Fluorescence measurements involve the detection of light emitted from a sample (a fluorophore) that has been excited by absorbing light from an incident beam. Figure 2b illustrates a typical fluorescence microfluidic platform. In detecting florescence, the cells or microparticles are tagged with fluorophores that absorb and emit light at known wavelength ranges. A beam of light is used to excite the tagged analyte in a microfluidic channel wherein the targeted analyte passes at a certain flowrate. The excited analyte subsequently emits light at a different wavelength. The emitted light can be isolated (such as by an optical filter) and used to quantify or image the analyte. Fluorescence measurements have high sensitivity and selectivity.
3. Chemiluminescence
Chemiluminescence measurements involve the detection of light released from excited molecules, ions, or atoms formed in a chemical reaction. Such reactions can be initiated on a microfluidic cartridge as part of the assay execution process. The intensity of emitted light is highly dependent on the number of reacting molecules and can be quantified against a dark background. This phenomenon provides a simple and highly sensitive means for chemical detection and has the advantage of not requiring a light source. An example of chemiluminescence measurement implemented on a microfluidic cartridge is illustrated in Figure 3.
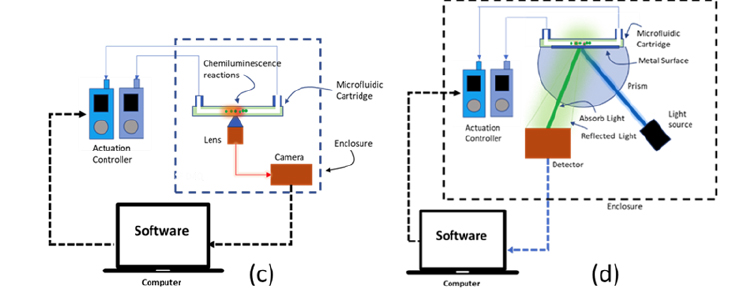
4. Surface Plasmon Resonance (SPR)
SPR measures a refractive index change caused by a sample coming in contact with a metal film. While the underlying physics are somewhat complex, the refractive index change is inferred by measuring changes in an angle at which the intensity of reflected light decreases sharply (due to propagating surface plasmons). SPR is the basis for many measurements involving the adsorption of a sample onto a metallic surface and is the fundamental principle behind a number of microfluidic sensors. In SPR biosensing, the change in refractive index is caused by the adsorption of analyte molecules on a metal surface that has been functionalized with probe molecules such as antibodies. An example of SPR implemented in a microfluidic system is shown in figure 3. SPR measurements have high sensitivity but require complex and expensive instrumentation.
Key Elements in Choosing an Optical System
1. What analytes to detect?
Understanding which analytes must be detected is crucial in selecting the right assay and optical detection method. It is advisable to review the published literature related to the target analytes as a reference.
2. What assay is most appropriate?
An assay capable of detecting the appropriate analyte must be selected. This will determine the workflow and the processes involved inside the microfluidic cartridge. The assay will dictate the complexity and the feasibility of the cartridge.
3. What detection method and sensor technology to use?
Once the assay is selected and you have an idea of a possible cartridge design based on the workflow, it is time to identify the optical system including the detection techniques and the sensor technologies to use. Consider the overall intended platform architecture including the limitations of the platform dimensions, as well as usability and human factors considerations.
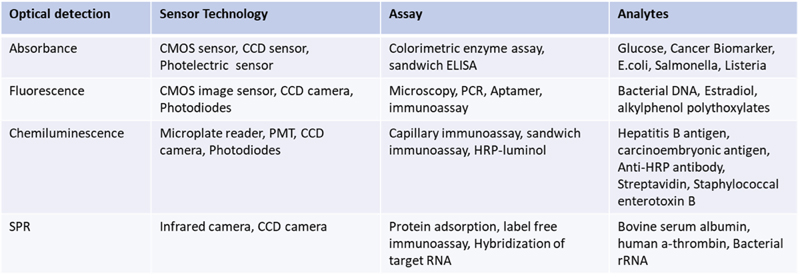
Table 1 summarizes typical examples of optical detection techniques, along with the applicable sensors, assay selections and target analytes for each. There are thousands of analytes to detect depending on the intended applications. Assay identification and development requires enormous time and effort, but when vetted using laboratory test data, integrating an assay to a microfluidic format with appropriate optical detection and sensors becomes much more feasible.
Conclusion
Appropriate optical detection systems are critical for the effective analysis of target analytes in a microfluidic format and the overall success of a microfluidic platform (i.e. diagnostic, analytical or research instrument).
This article presented recommendations for an optical imaging and detection techniques used in microfluidics and key elements in deciding which optical system to use.
The techniques presented herein are by no means exhaustive. Other detection techniques that can be implemented on a microfluidic cartridge include electrochemical detection, mechanical analysis, and spectroscopic methods (Raman, NMR and mass spectrometry). There are three important elements in selecting the appropriate detection means for a particular assay, (1) knowing the details of the assay and target analytes, (2) translating the assay to microfluidic format, and (3) selecting the proper detection technology.
Resources
- T. Miura, H. Mikami, A. Isozaki, T. Ito, Y. Ozeki, and K. Goda (2018) “On-chip light-sheet fluorescence imaging flow cytometry at a high flow speed of 1 m/s,” Biomed. Opt. Express 9, 3424-3433. https://doi.org/10.1364/BOE.9.003424
- Min, X., Fu, D., Zhang, J. et al. (2018) An automated microfluidic chemiluminescence immunoassay platform for quantitative detection of biomarkers. Biomed Microdevices 20, 91. https://doi.org/10.1007/s10544-018-0331-3
- Pennathur, S & Fygenson, D. (2008). Improving fluorescence detection in lab on chip devices. Lab on a chip. 8. 649-52. https://doi.org/10.1039/b805064n
- Pires, Nuno & Dong, Tao & Hanke, Ulrik & Hoivik, Nils. (2014). Recent Developments in Optical Detection Technologies in Lab-on-a-Chip Devices for Biosensing Applications. Sensors (Basel, Switzerland). 14. 15458-15479. https://doi.org/10.3390/s140815458
- Francis P.S. (2015) Chemiluminescence Detection. In: Li D. (eds) Encyclopedia of Microfluidics and Nanofluidics. Springer, New York, NY. https://doi.org/10.1007/978-1-4614-5491-5_216
- Kara B. Spilstead, Richard Alexander, Egan H. Doeven, Stephen J. Haswell, Neil W. Barnett, Paul S. Francis (2017) Evaluation of coloured materials in microfluidic flow-cells for chemiluminescence detection, Analytica Chimica Acta, Volume 968, Pages 66-73, ISSN 0003-2670. https://doi.org/10.1016/j.aca.2017.03.001
- Grillet, P. Domachuk, V. Ta’eed, E. Mägi, J. A. Bolger, B. J. Eggleton, L. E. Rodd, and J. Cooper-White, (2004) “Compact tunable microfluidic interferometer,” Opt. Express 12, 5440-5447 https://doi.org/10.1364/OPEX.12.005440