FDM/FFF (Fused Deposition Modeling/Fused Filament Fabrication)
FDM uses thermoplastic filament that is fed through a nozzle to create fine micro-scale strands, deposited side-by-side and layer-by-layer. This eventually builds a finished part. The part’s walls can be solid, but more commonly have variable in-fill density, which can make for lighter parts. This can be anything from parallel layers of material to stronger, honeycomb-like internal structures, and is managed through software with input on density and dimensions. Since engineering-grade plastics can be used, the parts can exhibit strength properties approaching injection molded or machined plastic parts. Also, because thermoplastics are reflowable, heated inserts can be used for fastening. This increases part application potential due to the strength of both the parts and fastening points. However, surface quality is generally an issue due to the cosmetic lines/layers on surfaces and support witness points created during fabrication. Certain materials, such as ABS, can be manually polished with abrasives; however, other materials, like PLA, cannot. It is important to consider the appearance requirements of the final state of the 3-D print and any post-processing techniques you wish to employ.
The build direction for the print is also important to consider, as it affects both function and appearance. Certain orientations will produce a more pronounced layered finish, such as contours. The build direction can also influence the strength of the part (tension, compression, bending, etc.), the amount of additional support material used, and the level of detail or amount of functionality in a given feature. Another parameter to consider is shrinkage of parts. Certain materials with higher processing temperatures, like ABS, will exhibit shrinkage, especially when used for larger parts. With these materials, this creates design constraints such as a required lower in-fill (reduced density) of material in larger parts.
Depending on the capabilities of an FDM printer and the functional material used, water soluble support structures exist. This can help when complex shapes and cosmetic supported surfaces are required in the final part. The soluble material is used to support the part being built and, after the build is complete, the composite part can be soaked until the support structures simply melt away. Some manual application with tools to scrape away remaining support structures is necessary; however, soluble support material removal can be automated with the use an ultrasonic or circulation tank.
SLA/SL (Stereolithography)
SLA employs a photo-polymer resin bath, platform and ultraviolet (UV) laser beam to create parts on a micro-scale, layer-by-layer. These parts can be completely solid or hollow. Solid parts have the benefit of strength and rigidity but end up costing more in materials. Hollow parts reduce the required material and lower the part cost; however, they require drainage features to allow for entrained resin to escape, which must be considered in the initial stages of design. SLA resins form thermoset plastics when cured, which cannot be reflowed and therefore heated inserts cannot be used for fastening. However, alternative insert methods can be employed, such as helical or press fit inserts.
High resolution builds using SLA can yield finished parts that show little evidence internally or externally of layering, unlike FDM. Standard post-processing by vendors (blasting) creates a matte finish on the parts, which is suitable for most uses. However, when optical clarity is required, vendors can provide additional processing to produce surfaces that emulate polycarbonate or acrylic, allowing for optical applications such as lenses or parts used to visualize internal flow of fluids. This can also be achieved by considering build direction and the flatness of a surface, as side walls and top surfaces without contours or support structures will be optically clear (of course, without blasting specified when ordering).
There are a wide range of SLA materials that can emulate the strength of traditionally manufactured materials, from polypropylene to die-cast aluminum. Metal-emulating parts built with SLA printers require significant post processing that increases cost of parts, but results in high detail with mechanical properties approaching that of metals. Finally, because the curing process employs a laser beam, ultra-fine resolution can be achieved. Vendors guarantee layers formed as fine as 0.001” (0.025mm), with features as small as 0.002” (0.050mm), for certain fine resolution material choices. More general-use materials with enhanced mechanical properties offer layers of 0.002” (0.050mm), with features of 0.005” (0.125mm).
SLS/DMLS (Selective Laser Sintering/Direct Metal Laser Sintering)
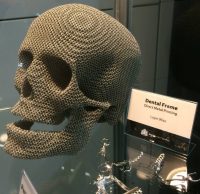
SLS comprises a high-powered, pulsed CO2 laser, powdered material (plastics, glass, ceramics), and a bed-roller assembly. Powdered material is applied by the roller in thin sheets. Sweeps of the laser then sinters (or fuses) together material line-by-line, creating parts with up to 100% in-fill density. DMLS employs the same process, but instead uses applicable metals (aluminum, titanium, stainless steel, etc.). An advantage of the SLS process is that it allows for the highest strength plastic parts across all three processes. Engineering-grade plastics with high stiffness and strength along with the potential for carbon-fibre or glass-fibre in-fill result in extremely tough SLS parts. These parts can be employed in the most demanding of applications, with functional features such as snap fits providing assemblies with mechanisms that reduce the need for separate fastening components.
Standard surface finish is a matte/sandy texture due to the fabrication process, but this can be polished or blasted to a desired final finish. Repeatability across a small volume of parts is high when compared to machined components, as parts are created on the same bed.
A distinct advantage of SLS over FDM or SLA is that since parts are supported by unsintered surrounding material, there is no need for support structures for features. This allows a consistent surface without the need for post-processing to remove supports. Parts can also be made within parts, which allows for the exploration of alternative designs; the only caveat here is that unsintered material within parts must be removed after fabrication. This can be accomplished by adding drain holes or the equivalent to access void spaces.
Process Comparison | |||
Process | FDM | SLA | SLS |
Part Quality | Low to Medium | High | High |
Plastic Type | Thermoplastic | Thermoset | Thermoplastic |
Surface | Layered finish | Matte to smooth/clear finish | Matte to smooth/clear finish |
Transparency | Opaque material | Opaque to transparent material | Opaque material |
Mechanical Strength | High tensile strength | Brittle | High overall strength |
Layer Thickness | 0.125–0.5 mm | 0.025–0.05 mm | 0.10–0.25 mm |
Support Structure | Needed | Needed | Not needed |
Airtight | No | Yes | Yes |
Chemical Resistance | Not suitable for fluids | Not defined | High |
Materials Available | ABS, PLA, nylon, polycarbonate, polyamide, polystyrene, elastomers | Photocuring resins | Polystyrene, polyurethane, nylon, aluminum, titanium, stainless steel, cobalt chrome, inconel |