The hype surrounding the potential of 3D printed medical devices is limitless, from prosthetic arms to surgical models to bioprinted organs. But in reality, there are material constraints for implantable applications. For the technology to move forward, the range of biomaterials used in 3D printed needs to be expanded. “Every application will need its own material development, and that’s not a trivial thing,” said David Kolesky of the Lewis Lab (Harvard University) at the Inside 3D Printing Conference and Expo in New York City last month.
A variety of biomaterials have been explored for use in 3D printing, especially in the research space. Nozzle-based systems have used hydrogels and polymer filaments, polyethylene glycol, collagen, and polylactic acid (PLA). Laser/light polymerization systems have incorporated polypropylene fumarate, PLA, and bioactive glass. Laser sintering/melting systems have used PLA, polyvinyl alcohol, titanium, bioactive glass, and polyetheretherketone (PEEK). These are just a few examples.
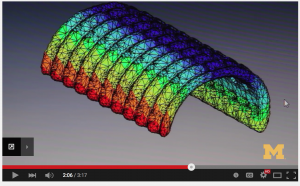
While industry is beginning to investigate this broad range of materials, adapting them for 3D printed applications is still a major challenge, according to Scott Hollister, Ph.D., renowned surgeon and professor of biomedical engineering and mechanical engineering at the University of Michigan. Hollister, who also spoke at Inside 3D Printing, is most well known for saving the life of a three-month-old by implanting a 3-D printed tracheal splint.
According to Leslie Oliver Karpas, CEO of Metamason, much of the investment dollars are going into the 3-D printers themselves. There is an over-focus on the machines and not enough emphasis on the actual materials used in the 3D printing process.
Scott Hollister, surgeon and professor of biomedical engineering and mechanical engineering at the University of Michigan, is renowned for developing a 3D printed tracheal splint for a baby. Several factors need to be considered when exploring the future of biocompatible materials for 3D printed implants. The design must take into account the ability to mimic anatomic geometry and specific properties such as porosity, as well as provide biologically active and load-bearing capabilities for scaffolds (think tissue engineering and regenerative medicine, where the facilitating tissue regrowth is fundamental). “[We] need to use materials conducive to keeping cells alive, but they tend to be soft,” said Hollister. “We need to better understand tissue properties to create materials that are more biocompatible.” Available materials need to have a range in compliance, from soft and stretchy to hard and stiff.
Changing the Engineering Mindset
While 3D printing is a structural advance, engineers and designers need to understand the formulation of materials to advance the field. This involves not just a refinement in materials, but also a change in mindset.
“A new mentality has to be developed in the way you formulate 3D printing compositions. We need to find more materials that meet the [requirements of the] immune system and the biocompatibility requirements, and at the same time, meet the engineering requirements of the extruder,” says Peter Gabriele, vice president of R&D at Secant Medical, Inc. “That’s going to take someone who is knowledgeable not only on the polymer chemistry side, but also someone who is willing to learn that there’s a whole host of new materials that has to be evaluated.”
Bringing in a new biomaterial to 3D printing is no different from bringing a new biomaterial to the rest of the medical device world, according to Gabriele. The biomaterial has to be tested in the context of device under ISO 10993 Biological Evaluation of Medical Devices. Thus the constraint on 3D printing is equal to that of bringing a new bioresorbable material to the market, for example.
“When you look at this entire realm of 3D printing, it can be reduced down to a very simple concept—all it is high-definition, three-dimensional extrusion,” says Gabriele. The process of 3D printing involves pushing a material through a very small hole, which introduces constraints related to viscosity and melt points, the thermal behaviors required to process the material must be taken into account. For example, the material passing through the extruder must not require such a high-melt temperature that it causes oxidation of the material. “The materials of construction of the future have to somehow be able to flow and set up without any kind of interference with the biological process from the crosslink agent, the curing mode, or whatever it might be that subjects the scaffold to degradation processes that by themselves the by-products affect the tissues,” says Gabriele.
As industry looks to the future of 3D printed medical devices, the adaptation of more biocompatible materials must be further developed. Manufacturers will need to consider the desired tissue-like properties for implants and whether the biomaterial, when processed, will be an appropriate form for the machine. Quality control, reproducibility, and demonstrated improved patient outcomes will be key factors from FDA’s perspective. Last fall, the agency held a public workshop on additive manufacturing of medical devices, but it is still learning about the challenges and technical considerations of 3D printed technology.